Strange Illuminations at the PSRs: A Study of the Dark Regions of the South
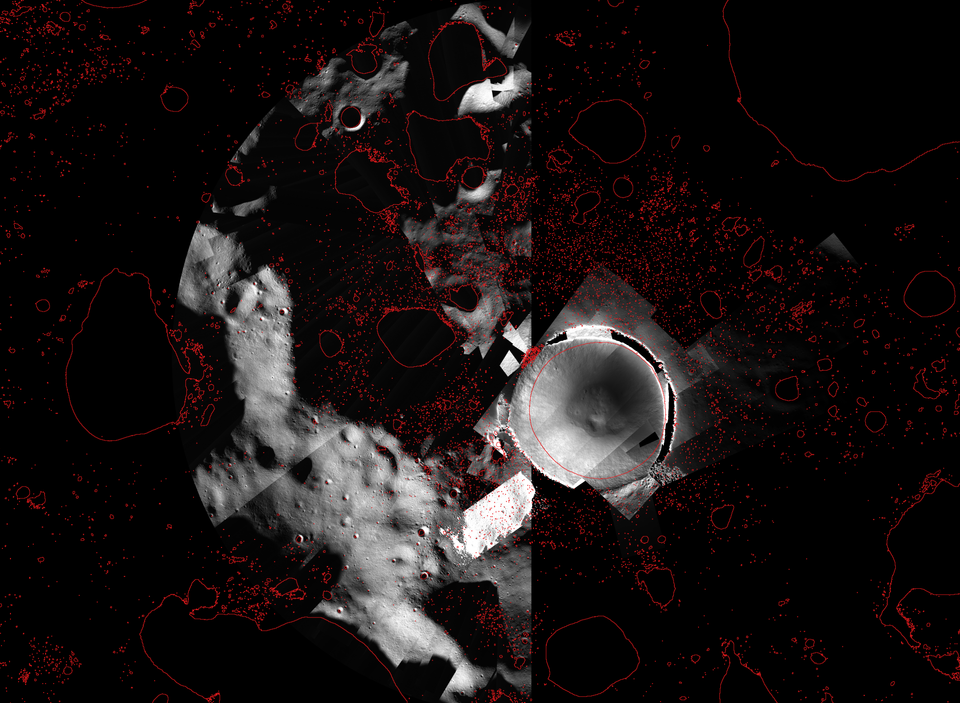
Over the last two decades, the lunar South Pole has emerged as a focal point for great scientific inquiry, driven by its potential to support future human missions and the possibility of establishing a permanent base on the Moon. This forward-thinking approach aims to lay the groundwork for more ambitious explorations such as Artemis.
Exploring the Moon isn't just about understanding its history; it's also crucial for planning our future in space. Many have expressed their desire for NASA to continue leading this effort. Frankly – it's evident that other spacefaring nations are rapidly advancing, narrowing the gap in exploration capabilities. I believe that future endeavors will necessitate a collective and collaborative approach, fostering a synergy that will benefit the advancement of space exploration.
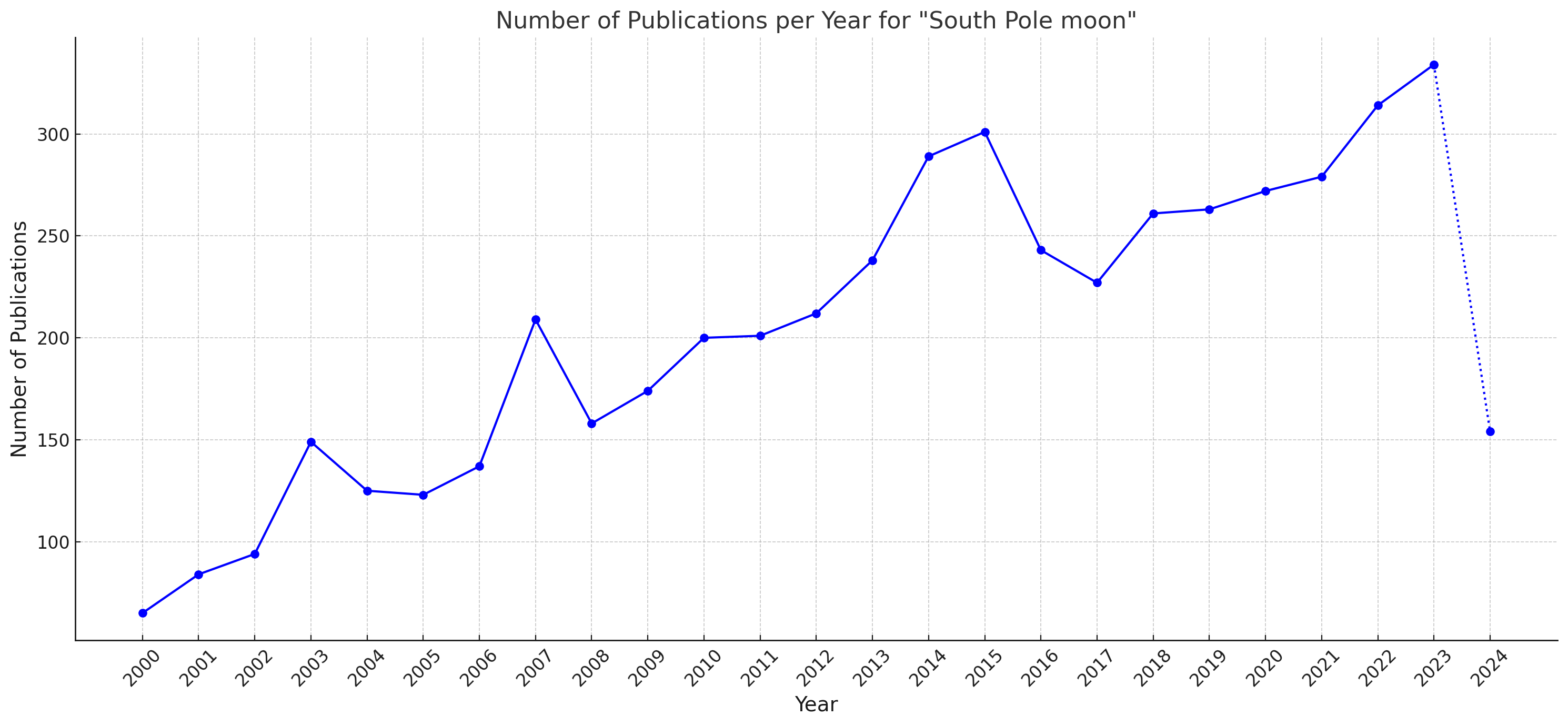
Permanently Shadowed Regions
The term "Permanently Shadowed Regions" (PSRs) refers to areas on the Moon that never receive direct sunlight due to the low obliquity of the Moon (1.54°). The PSRs at the South Pole are particularly intriguing because they are theorized to contain water ice and are shaded more than those at the North Pole, making them a key focus for research and exploration [8].
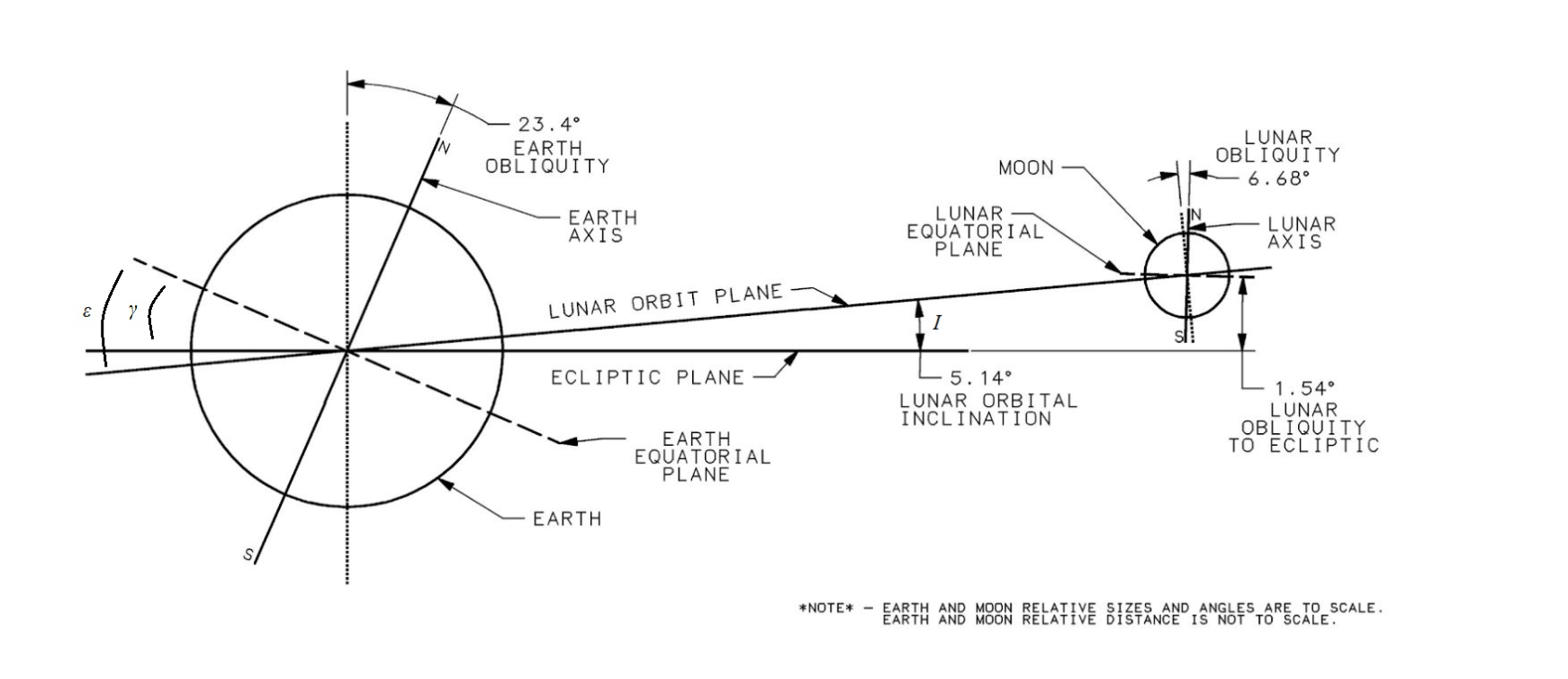
Water, a volatile substance on the lunar surface, is especially known to be present and is identified as the most stable compared to other naturally occurring volatiles that might have been released at any point. The quantity of water potentially lost since the lunar surface conditions evolved to the current state is very small.
As a result, the current levels of lunar ice are believed to closely reflect the total amount of water historically present on the lunar surface [7]. Naturally, these regions are of significant interest in planetary science and exploration. The believed water is thought to be trapped in the lunar soil, possibly deposited by impacts from space millions of years ago. This makes them not just important for scientific study but also potential sources of water for future lunar missions. The potential for extracting this ice signifies the importance of studying and exploring PSRs.
Cold Trapping
Cold-trapping is a process where certain regions can trap and freeze volatile substances like water vapor due to their extremely low temperature. Because of the lack of direct sunlight, these PSRs remain cold and have extremely low temperatures. Water sublimation (water to gas) is highly non-linear and slow at low temperatures. Hence, the probability of frozen water.
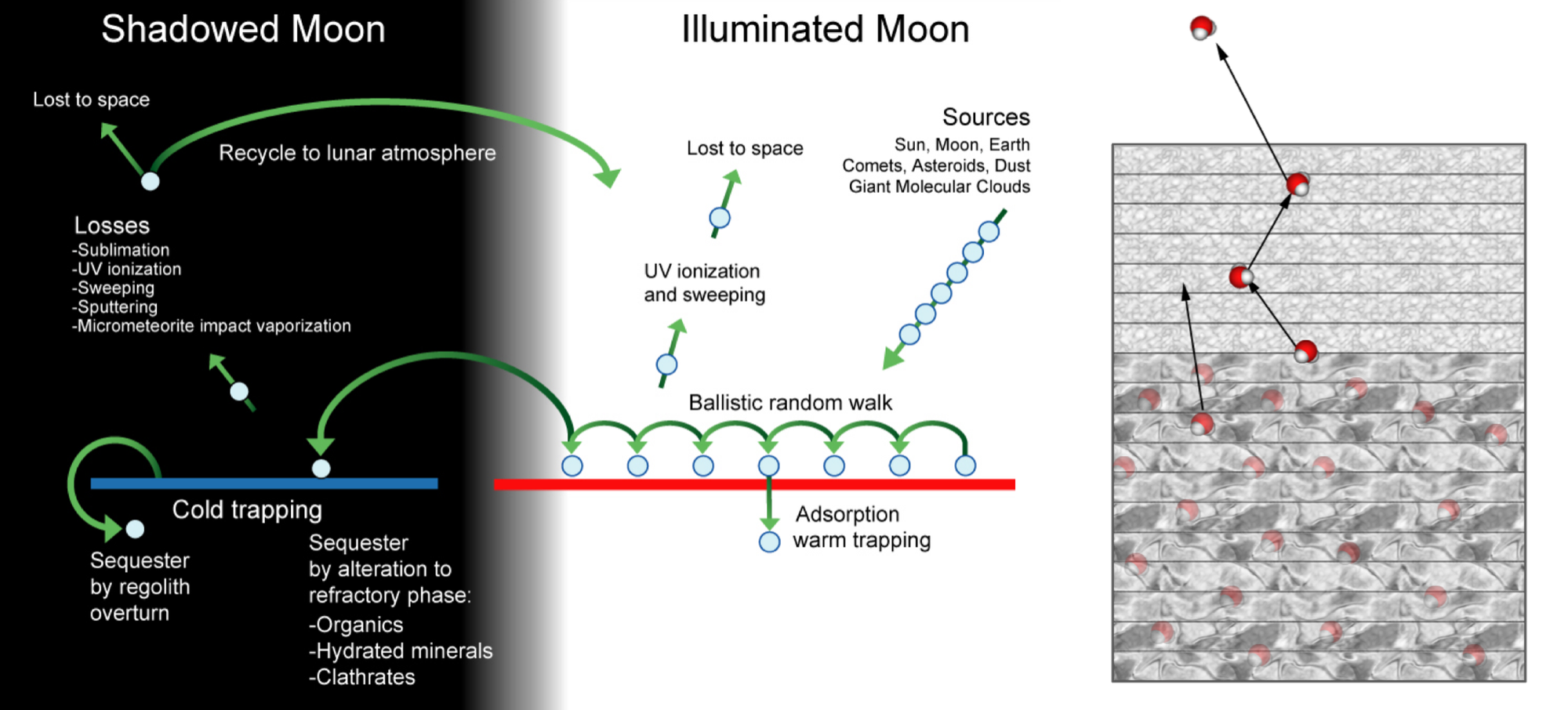
Temperature (C / K) | Area (km²) |
---|---|
-163 / 110 | 3.73 × 10^4 |
-162 / 111 | 3.79 × 10^4 |
-161 / 112 | 3.86 × 10^4 |
-160 / 113 | 3.92 × 10^4 |
-159 / 114 | 3.98 × 10^4 |
-158 / 115 | 4.04 × 10^4 |
Seasonal Winter Cold‐Trapping Area in South Polar Region for Threshold Temperatures [11]
Temperature Variations at PSR
The temperature at Permanently Shadowed Regions (PSRs) and the corresponding cold trapping depend on several factors, including topographical facets and the thermal conductivity of the regolith. One such factor is the phenomenon known as Secondary Illumination [1], I'll talk about it in the next sections.
From the Diviner observations [11], the probabilities of water trapping increase approximately 3 times during the winter. Moreover, the PSRs that cast double shadows due to multiple light scattering within the PSRs are observed to have lower temperatures and thereby increased chances of water deposits [12].
In the craters that exhibit double shadows, the heat sources are the thermal radiation due to the multiple light scattering that is the secondary form of "Illumination" inside the crater and is dependent on the
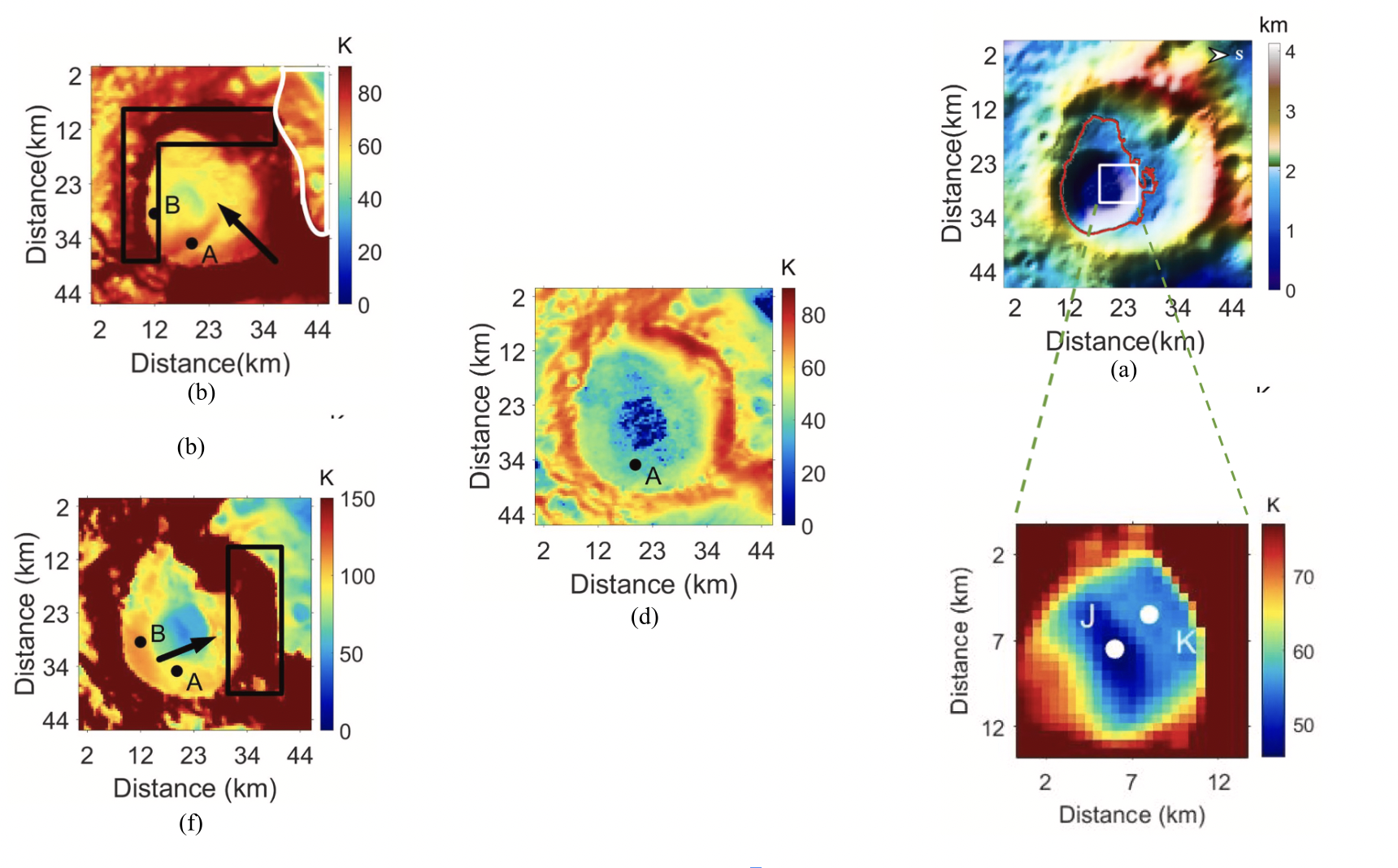
Future missions to the South Pole like LUPEX, VIPER are planned to do in-situ studies at the regions thought to have contain the water deposits.
Imaging and cataloging PSRs
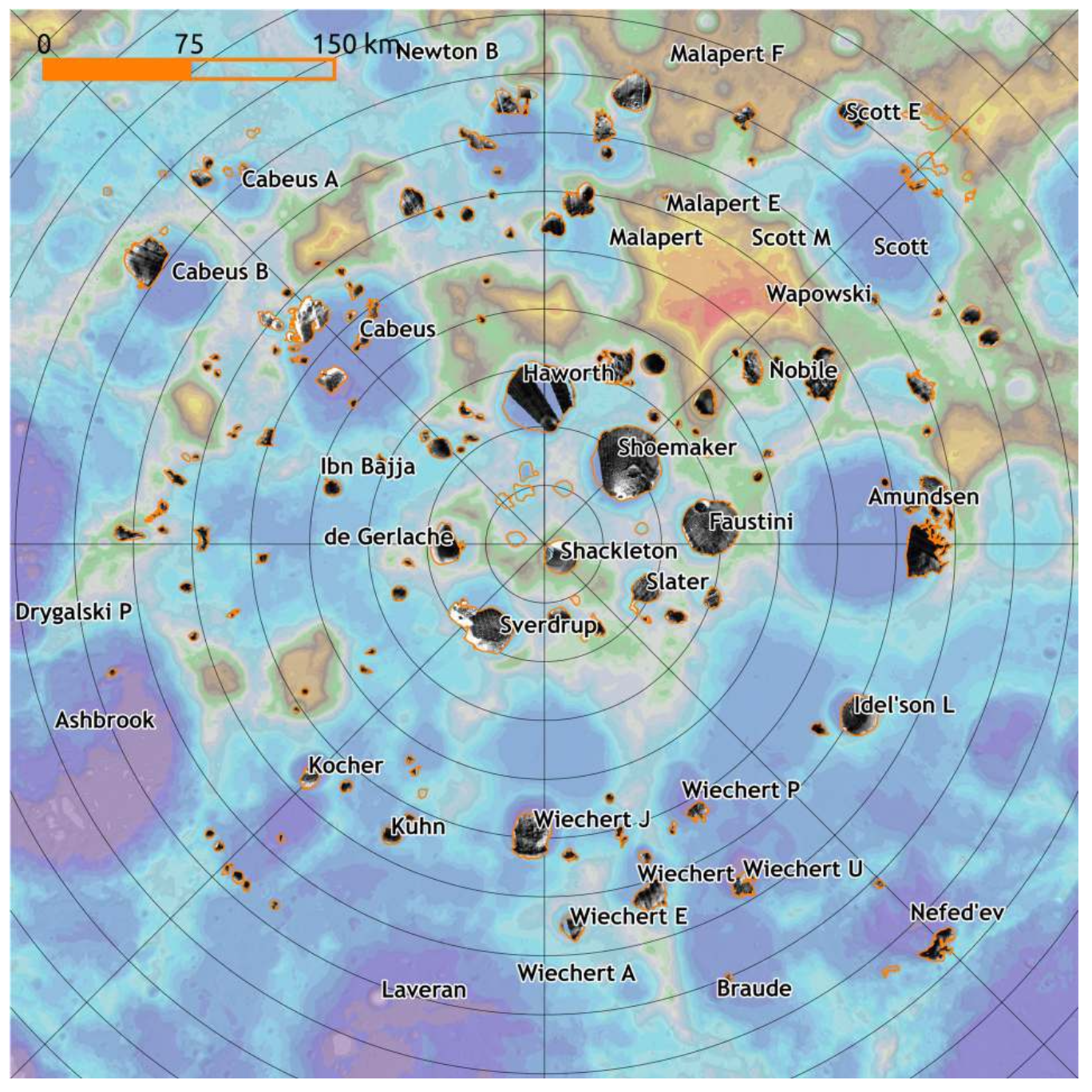
It is now quite clear that imaging locations in low illumination conditions is challenging. Prior to the ShadowCam [14], the LRO team utilized the NAC to image PSRs. NAC images were captured using increased exposure times, and to enhance the SNR, the crosstrack-elongated pixels were binned to create a single pixel. Further calibration was performed using lronaccal
and lronacecho
routines. Ultimately, these images were orthorectified and stretched (2-98%) to create a final PSR NAC product.
All PSRs spanning areas greater than 10 km² have been cataloged in the PSR Atlas [5], each receiving a unique identifier. The atlas currently encompasses 324 PSRs across both the South and North Polar regions. Each PSR is assigned a unique ID; the ID begins with the first two characters indicating the polar region (NP for North Pole or SP for South Pole), followed by the centroid latitude, and then the centroid longitude. The values are delimited by underscores, with the latitude accurate to up to six digits, and the longitude to seven digits.
For eg: SP_871460_0840750
indicates a PSR in the South Pole region with the center 87.146 S, 84.075 E
The Physics of Secondary Illumination
In the preceding sections, I have explored the phenomenon of double shadows and their occurrence despite the absence of direct sunlight. This effect can be attributed to the topographical features within certain regions, leading to "secondary" illumination effects caused by light scattering off the crater's interior facets.
![Illustration showing primary illumination (yellow) and secondary illumination (blue) formed due to a single bounce of light from the topographic facet [1]](https://moonandbeyond.blog/content/images/2024/03/image-15.png)
The above illustration explains the scattering of light rays, emphasizing the "yellow" areas directly illuminated by solar incidence. The "blue" areas represent regions illuminated by rays scattered from the "yellow" areas. Here, the illuminated parts of the crater, typically the upper regions of the crater wall, contribute as the "emitters," while the Permanently Shadowed Regions (PSR) act as the "receivers".
For clarity, the illustration simplifies this to a single bounce of light, though, in reality, multiple bounces are possible. An observer-independent concept, introduced in [1], has been applied to elucidate these illumination phenomena at Shackleton [2] and extended to investigate potential locations for the Artemis missions [3].
In the case of secondary irradiation (Ii,j 1,2)—where the facets act as receivers—there is a strong correlation between the positions and orientations of the topographical facets and the Primary illumination (Ij,k 0) which serves as emitters.
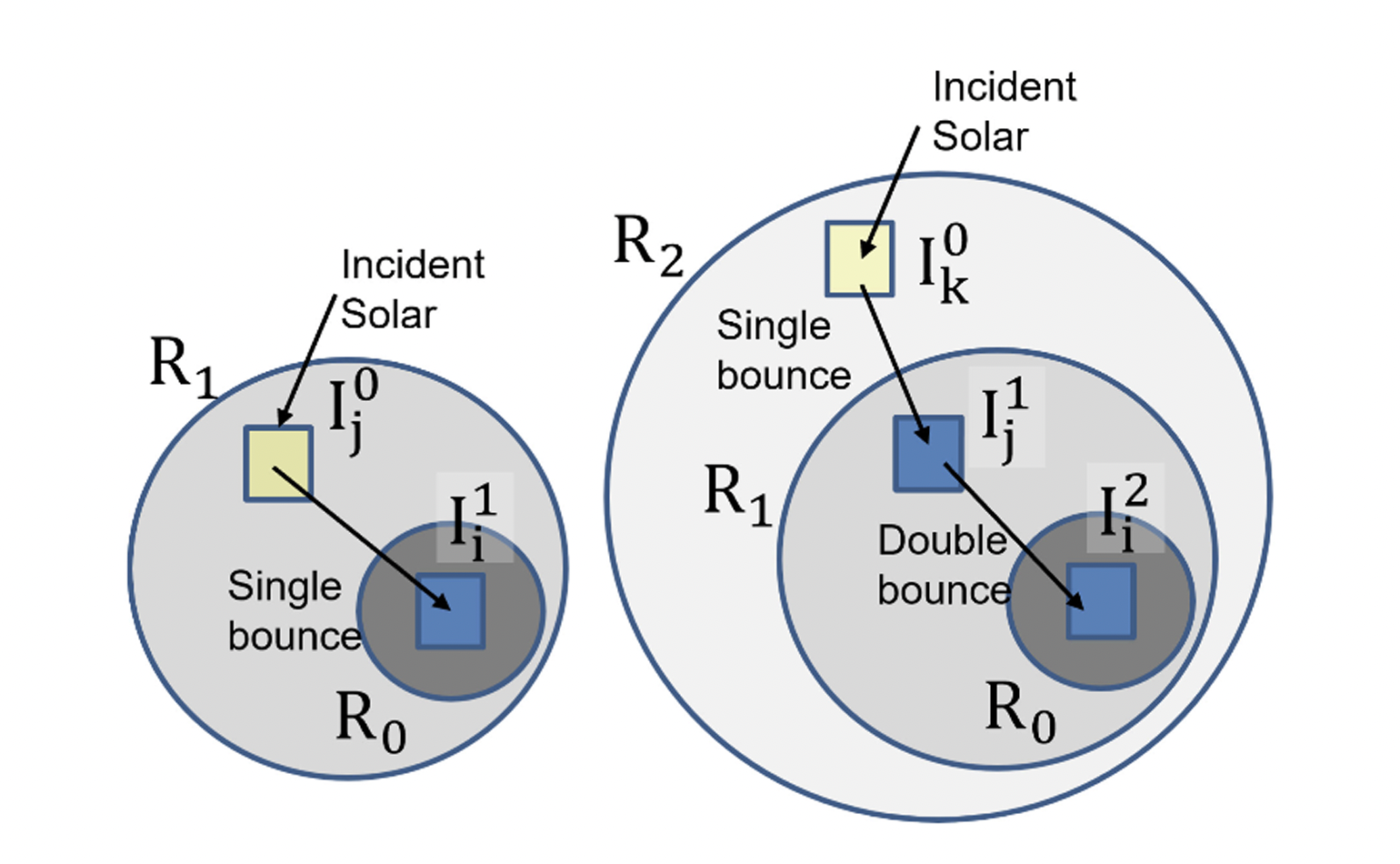
I will not delve into the specifics of this model; the detailed explanation of the simulation model outlined above is provided in Sections II, and III in [1].
From the simulation results, a correlation between the simulated illuminated PSR and NAC images showed moderate agreement, with factors like stray light contamination and local photometric properties impacting the correlation negatively. Initial experimental results further validated the effectiveness of this methodology.
![A Comparision of NAC Long exposure image and Simulated image based on Secondary illumination from [1] of Ibn Bajja Crate](https://moonandbeyond.blog/content/images/2024/03/image-34-1.png)
The approach for the computational estimation of secondary irradiance within lunar Permanently Shadowed Regions (PSRs), independent of an observer's position. While the method demonstrates a commendable capability in aligning simulated images with those obtained from LROC NAC long exposure captures—particularly in reflecting the impacts of seasonal insolation shifts and topographical nuances—an assessment suggests a refinement. Specifically, the methodology's robustness in varying lunar environmental conditions and its scalability for comprehensive lunar exploration missions could benefit from further exploration.
Nevertheless, the technique holds significant potential. It lays a foundational step towards enriching our pre-visit knowledge of lunar surfaces, particularly in PSRs, and potentially improving the quality and efficacy of lunar imaging.
In my next few posts, I'll talk about how these methods help us understand Shackleton and areas chosen as future landing missions.
[1] P. Mahanti, T. J. Thompson, M. S. Robinson, and D. C. Humm, "View Factor-Based Computation of Secondary Illumination Within Lunar Permanently Shadowed Regions," IEEE Geoscience and Remote Sensing Letters, vol. 19, 2022.
[2] P. Mahanti, M. S. Robinson, D. C. Humm, R. V. Wagner, N. M. Estes, and J.-P. Williams, "Preliminary Characterization of Secondary Illumination at Shackleton Crater Permanently Shadowed Region from ShadowCam Observations and Modeling," J. Astron. Space Sci., vol. 40, no. 4, pp. 131-148, 2023. DOI: 10.5140/JASS.2023.40.4.131.
[3] P. Mahanti, J.-P. Williams, M. S. Robinson, R. Wagner, E. Mazarico, M. Henriksen, H. Brown, and N. Kumari, "Dynamic Secondary Illumination in Permanent Shadows within Artemis III Candidate Landing Regions," The Planetary Science Journal, vol. 5, no. 3, 2024. DOI: 10.3847/PSJ/ad1b50.
[4] H. Bernhardt, M. S. Robinson, and A. K. Boyd, "Geomorphic map and science target identification on the Shackleton-de Gerlache ridge," Icarus, vol. 379, 2022, Art. no. 114963, doi: 10.1016/j.icarus.2022.114963.
[5] PSR Atlas http://lroc.sese.asu.edu/psr
[6] SER Data Portal https://data.ser.asu.edu/
[7] Watson, Kenneth M., Bruce C. Murray and Harrison S. Brown. “The behavior of volatiles on the lunar surface.” Journal of Geophysical Research 66 (1961): 3033-3045.
[8] E. M. Shoemaker, M. S. Robinson, and E. M. Eliason, "The South Pole Region of the Moon as Seen by Clementine," Science, vol. 266, no. 5192, pp. 1851-1854, 1994, doi: 10.1126/science.266.5192.1851.
[9] Goldreich, Peter. "History of the Lunar Orbit". Reviews of Geophysics, Vol 4. November 1966.
[10] Paul G. Lucey, "The Poles of the Moon",DOI: 10.2113/gselements.5.1.41
[11] Williams, J.‐P., Greenhagen, B. T., Paige, D. A., Schorghofer, N., Sefton‐Nash, E., Hayne, P. O., et al. (2019). "Seasonal polar temperatures on the Moon. Journal of Geophysical Research: Planets, 124, 2505–2521." doi:10.1029/2019JE006028
[12] Z. Yin, N. Liu, Y.-Q. Jin, "Simulation of the temperatures in the permanently shadowed region of the Moon's south pole and data validation," Icarus, vol. 411, 115917, 2024. doi:10.1016/j.icarus.2023.115917
[13] E. Cisneros, A.K. Boyd, H.M. Brown, A.A. Awumah, A.C. Martin, K.N. Paris, J. Leland, R.Z. Povilaitis, R. Hoppe, L.M. Davis, N.M. Estes, M.S. Robinson, and the LROC Team "Lunar Reconnaissance Orbiter Camera Permanently Shadowed Regions Atlas" July 6, 2018
[14] M. S. Robinson, P. Mahanti, L. M. Carter, B. W. Denevi, N. M. Estes, M. A. Ravine, E. J. Speyerer, and R. V. Wagner, "ShadowCam – Seeing in the Dark," in Proc. European Planetary Science Congress 2017, vol. 11, EPSC2017-506, 2017.
Member discussion